Imagine a world without sunlight. Life as we know it wouldn’t exist. Sunlight provides energy to our planet, and plants have evolved a fascinating process called photophosphorylation to convert this light energy into chemical energy. In this article, we will explore the intricate mechanism of photophosphorylation and delve into the secrets behind the conversion of sunlight into usable energy.
Photophosphorylation, the captivating process of sunlight-energy conversion, is a fascinating source of knowledge, unraveling the secrets behind the transformation of sunlight into chemical energy. Photophosphorylation is a complex biological process that occurs in the cells of photosynthetic organisms, such as plants, algae, and cyanobacteria. It involves the conversion of light energy from the sun into chemical energy in the form of ATP (adenosine triphosphate). This energy-rich molecule is essential for various cellular activities and drives the synthesis of organic compounds required for growth and development.
The Importance of Sunlight-Energy Conversion
Sunlight is the ultimate source of energy for life on Earth. It provides the energy needed for photosynthesis, the process by which plants and other photosynthetic organisms convert carbon dioxide and water into glucose and oxygen. Photophosphorylation is an integral part of photosynthesis, enabling the transformation of light energy into a usable form that sustains life on our planet.
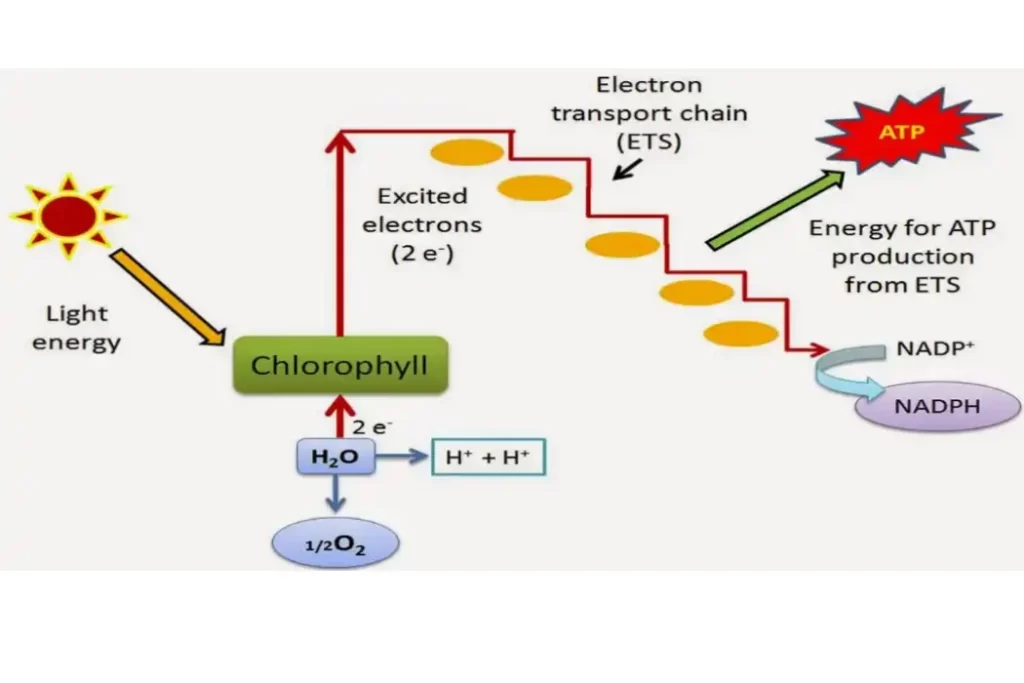
The Basics of Photophosphorylation
Light Absorption and Pigments
Photosynthetic organisms contain specialized pigments, such as chlorophyll and carotenoids, which absorb light energy. These pigments are located in specific structures called photosystems, which are embedded within the thylakoid membranes of chloroplasts. When light is absorbed, it excites the electrons within the pigments, initiating the process of photophosphorylation.
Photosystems: The Powerhouses of Photophosphorylation
Photosystems are multi-protein complexes that facilitate the capture and transfer of light energy. There are two types of photosystems: Photosystem I (PSI) and Photosystem II (PSII). Each photosystem consists of pigments, reaction center complexes, and electron carriers. PSII functions first in the light-dependent reactions of photosynthesis, followed by PSI.
Electron Transport Chain
As the excited electrons move through the photosystems, they enter an electron transport chain. This chain consists of protein complexes and electron carriers that facilitate the flow of electrons. The energy released during this electron transfer is used to pump protons (H+) across the thylakoid membrane, creating a proton gradient.
ATP Synthesis
The proton gradient generated by the electron transport chain powers an enzyme called ATP synthase. This enzyme utilizes the potential energy of the proton gradient to convert ADP (adenosine diphosphate) and inorganic phosphate (Pi) into ATP. ATP is the primary energy currency of the cell and is utilized in various cellular processes.
Types of Photophosphorylation
Non-Cyclic Photophosphorylation
Non-cyclic photophosphorylation is the most common pathway of photophosphorylation. It involves both PSII and PSI, and it generates ATP, NADPH (nicotinamide adenine dinucleotide phosphate), and oxygen. The excited electrons from PSII are transferred to PSI, and during this process, water is split, releasing oxygen as a byproduct.
Cyclic Photophosphorylation
Cyclic photophosphorylation is an alternative pathway that occurs in some photosynthetic organisms. It involves only PSI and generates ATP without the production of NADPH or oxygen. The excited electrons from PSI are cycled back to the electron transport chain, creating a continuous flow of electrons and ATP synthesis.
Factors Affecting Photophosphorylation
Several factors influence the efficiency of photophosphorylation in photosynthetic organisms. These include:
Light Intensity
The intensity of light directly affects the rate of photophosphorylation. High light intensity can lead to photodamage and the production of reactive oxygen species, while low light intensity may limit the availability of light energy for the process.
Temperature
Temperature plays a crucial role in photophosphorylation. Extreme temperatures can disrupt the structure and function of photosynthetic pigments and enzymes, affecting the overall efficiency of the process.
Availability of Water
Water is essential for photophosphorylation as it provides the electrons needed to replace those lost during the light reactions. Insufficient water availability can impair the process and limit ATP production.
Concentration of CO2
The concentration of carbon dioxide (CO2) influences the rate of photosynthesis and, consequently, photophosphorylation. Inadequate CO2 levels can lead to reduced ATP synthesis and overall productivity.
Photophosphorylation in Photosynthetic Organisms
Plants
Plants are the most well-known photosynthetic organisms. They utilize photophosphorylation to convert light energy into chemical energy, allowing them to synthesize glucose and other organic compounds required for growth, reproduction, and defense against environmental stressors.
Algae
Algae encompass a diverse group of photosynthetic organisms, ranging from single-celled microalgae to large multicellular seaweeds. They play a crucial role in aquatic ecosystems and are also used in various industries, such as biofuel production and wastewater treatment.
Cyanobacteria
Cyanobacteria, also known as blue-green algae, are a group of photosynthetic bacteria capable of performing oxygenic photosynthesis. They played a significant role in shaping Earth’s atmosphere by releasing oxygen billions of years ago.
Applications of Photophosphorylation Research
Understanding the intricacies of photophosphorylation has numerous practical applications. Researchers are exploring ways to harness and optimize this process for sustainable energy production, such as artificial photosynthesis and bio-inspired solar cells. Moreover, studying photophosphorylation can provide insights into improving crop yields and developing more efficient photosynthetic organisms for various industrial purposes.
Challenges and Future Directions
While photophosphorylation is a remarkable process, there are still many unanswered questions and challenges ahead. Scientists continue to investigate the details of photophosphorylation and explore new avenues for enhancing its efficiency. The future holds exciting possibilities, such as genetic engineering approaches to improve photophosphorylation and the integration of artificial systems with natural processes.
Conclusion
Photophosphorylation is a captivating process that enables the conversion of sunlight into chemical energy in photosynthetic organisms. Through the coordinated interplay of pigments, photosystems, electron transport chains, and ATP synthesis, these organisms capture and utilize the energy of sunlight to sustain life on Earth. Understanding the secrets behind photophosphorylation opens doors to diverse applications and offers a glimpse into the fascinating realm of solar energy conversion.
FAQ on photophosphorylation
Q1: What is photophosphorylation?
Answer: Photophosphorylation is the process by which sunlight is converted into chemical energy, specifically ATP, in photosynthetic organisms.
Q2: How does photophosphorylation contribute to the production of oxygen?
Answer: During the process of photophosphorylation, water is split, releasing oxygen as a byproduct. This oxygen is vital for supporting aerobic respiration and maintaining the oxygen levels in our atmosphere.
Q3: Are there different types of photophosphorylation?
Answer: Yes, there are two main types of photophosphorylation: non-cyclic photophosphorylation and cyclic photophosphorylation. Non-cyclic photophosphorylation produces ATP, NADPH, and oxygen, while cyclic photophosphorylation is limited to ATP synthesis.
Q4: What factors affect the efficiency of photophosphorylation?
Answer: Several factors influence the efficiency of photophosphorylation, including light intensity, temperature, the availability of water, and the concentration of carbon dioxide (CO2).
Q5: Can photophosphorylation occur in non-photosynthetic organisms?
Answer: No, photophosphorylation is a process exclusive to photosynthetic organisms capable of harnessing light energy for ATP synthesis.
Q6: What are the practical applications of photophosphorylation research?
Answer: Photophosphorylation research has practical applications in the development of sustainable energy technologies, such as artificial photosynthesis and bio-inspired solar cells. It also aids in improving crop productivity and exploring the potential of photosynthetic organisms for various industrial uses.